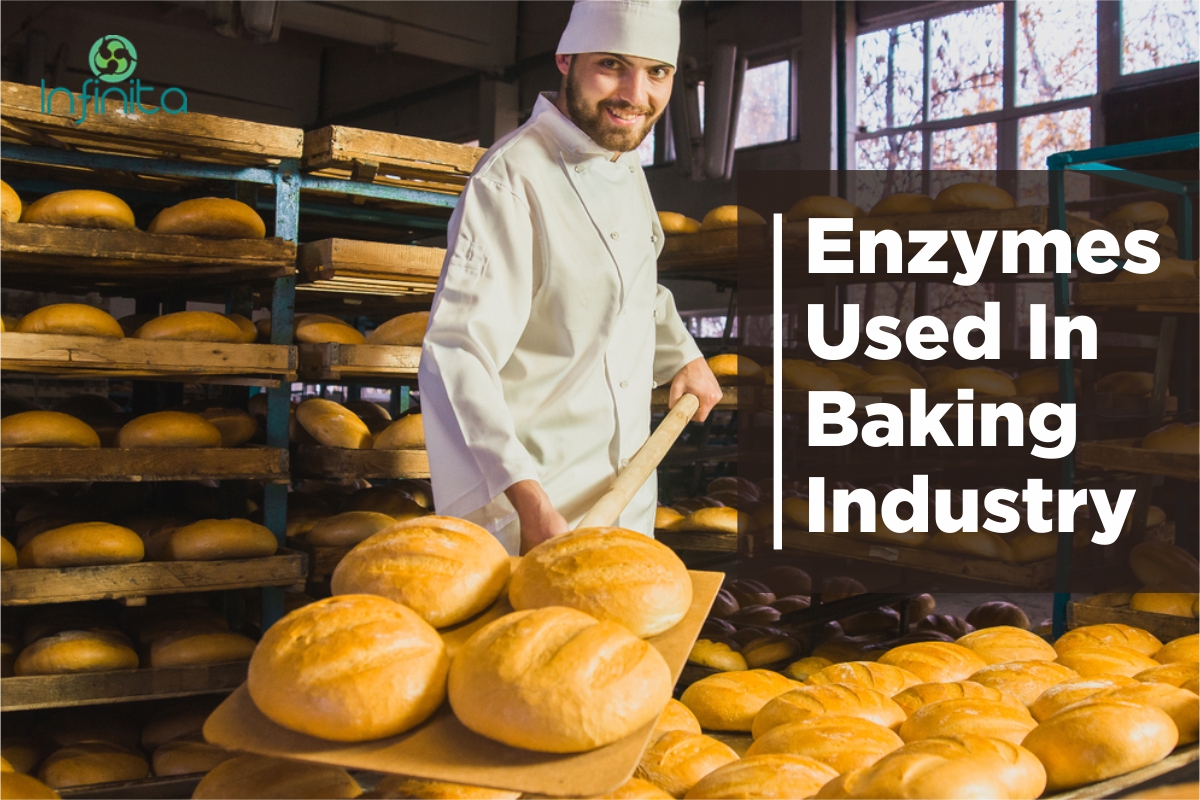
Enzymes Used In Baking Industry
Enzymes Used In Baking Industry
The use of enzymes dates from much longer than their ability to catalyse reactions was recognised and their chemical nature was known. The first completely enzymatic industrial process was developed in the years 1960. Starch processing, which is undertaken in two steps, involves liquefaction of the polysaccharide using bacterial α-amylase, followed by saccharification catalysed by fungal glucoamylase.
Developments in process technology allied to the use of recombinant techniques during the last decades allowed for considerably improved yields by fermentation increased stability and altered specificity and selectivity of enzymes. Those techniques thrust forward and are continuing to broaden the applications of enzymes in food technology and many different areas.
There are two scenarios regarding the use of enzymes, either the enzymes are used to convert the raw material into the main product, or the enzymes are used as additives to alter a functional characteristic of the product. In the first case, the enzymatic process is undertaken in optimised and controlled conditions to enhance the catalytic potential of the enzyme, whereas in the second situation it is more difficult to assure optimal conditions and to control the enzymatic reaction.
Bakery Enzymes Industry
The development of the bread process was an important event in mankind. An important aspect that contributed to the evolution of the baking market was the introduction of industrial enzymes in the baking process, where bakery enzymes represent a relevant segment of the industry.
Main Constituents Of Baked-Products
Baking is a common name for the production of baked goods, such as bread, cake, pastries, biscuits, crackers, cookies, pies and tortillas, where wheat flour is both the most essential ingredient and a key source of enzyme substrates for the product. Even though based on cereals other than wheat, baked goods such as gluten-free products or rye bread are also considered to be baked products.
Bread is usually made from wheat flour as raw material, which is a mixture of starch, gluten, lipids, non-starch polysaccharides, and enzymes. After flour, yeast, and water are mixed, complex biochemical and biophysical processes begin, catalysed by the wheat enzymes and by the yeast, characterising the dough phase. These processes go on in the baking phase, giving rise to bread.
Extra enzymes added to the dough improve control of the baking process, allowing the use of different baking processes, reducing process time, slowing-down staling, compensating for flour variability and substituting chemical additives.
Starch is the main component of products such as bread and other bakery goods and is added to different foods, acting as a thickener, water binder, emulsion stabiliser, gelling agent and fat substitute. It is the most abundant constituent and most important reserve polysaccharide of many plants, including cereals, occurring as intracellular, semi-crystalline granules. On a molecular level, its major components are the glucose polymers amylose and amylopectin.
Amylose is an essentially linear molecule, consisting of up to 6000 glucose units with α-(1,4)-glycosidic bonds. On the other hand, amylopectin is a highly branched polysaccharide constituted of short α-1,4 linked linear chains of 10–60 glucose units and α-1,6 linked side chains with 15–45 glucose units, containing on average 2 million glucose units.
Enzymes Used In Baked Products
Baking comprises the use of enzymes from three sources: the endogenous enzymes in flour, enzymes associated with the metabolic activity of the dominant microorganisms and exogenous enzymes which are added in the dough. The supplementation of flour and dough with enzyme improvers is a usual practice for flour standardisation and also as baking aids.
Enzymes are usually added to modify dough rheology, gas retention and crumb softness in bread manufacture, to modify dough rheology in the manufacture of pastry and biscuits, to change product softness in cake making and to reduce acrylamide formation in bakery products. The enzymes can be added individually or in complex mixtures, which may act in a synergistic way in the production of baked goods [60-62], and their levels are usually very low.
Hydrolases
Enzymes as technological aids are usually added to flour, during the mixing step of the bread-making process. The enzymes most frequently used in bread-making are the α-amylases from different origins. Amylases and other starch-converting enzymes The industrial processing of starch is usually started by α-amylases (α-1,4-glucanohydro‐ lase). Most of the starch-converting enzymes belong to the α-amylase family or family 13 glycosyl hydrolases (GH), based on amino acid se α-Amylases are endo-enzymes that catalyse the cleavage of α-1,4-glycosidic bonds in the inner part of the amylose or amylopectin chain. The end products of α-amylase action are oligosaccharides, with an α-configuration and varying lengths, and α-limit dextrins, which are branched oligosaccharides. These enzymes can be obtained from cereal, fungal, bacterial and biotechnologically altered bacterial sources.
Differences in the number of binding sites and location of catalytic regions determine the substrate specificity of α-amylases, the length of the oligosaccharide fragments released after hydrolysis and, consequently, the carbohydrate profile of the final product. The increased levels of reducing sugars lead to the formation of Maillard reaction products, intensifying bread flavour and crust colour. In addition, these enzymes can improve the gas-retention properties of fermented dough and reduce dough viscosity during starch gelatinisation, with consequent improvements in product volume and softness. Certain amylases are able to decrease the firming rate of bread crumb, acting as anti-staling agents.
Amylase-containing anti-staling products typically consist of bacterial or fungal α296 Food Industry amylases with intermediate thermostability. In this context, one of the most effective anti-staling amylases is the Bacillus stearothermophilus maltogenic α-amylase. The anti-staling action of amylases has been attributed to the modified retrogradation behavior of the hydro‐ lysed starch. Yet, other researchers describe the effect of the interference of the low molecular weight dextrins with starch-starch and/or gluten-starch interactions.
Proteases
Proteases can be subdivided into two major groups according to their site of action: exopeptidases and endopeptidases. Exopeptidases cleave the peptide bond proximal to the amino or carboxy termini of the substrate, whereas endopeptidases cleave peptide bonds distant from the termini of the substrate. Most of the proteolytic activity of wheat and rye flours corresponds to aspartic proteases and carboxypeptidases, which are both active in acid pH. Additionally, aspartic proteases of wheat are partly associated with gluten. Nevertheless, the proteolytic activity of sound, ungerminated grain is normally low.
Proteases are used on a large commercial scale in the production of bread, baked goods, crackers, and waffles. These enzymes can be added to reduce mixing time, to decrease dough consistency, to assure dough uniformity, to regulate gluten strength in bread, to control bread texture and to improve flavor. In addition, proteases have largely replaced bi-sulfite, which was previously used to control consistency through reduction of gluten protein disulfide bonds, while proteolysis breaks down peptide bonds. In both cases, the final effect is a similar weakening of the gluten network. In bread production, a fungal acid protease is used to modify mixtures containing high gluten content. When proteases are mixed in the blend, it undergoes partial hydrolysis becoming soft and easy to pull and knead. Proteases are also frequently added to dough preparations. These enzymes have a great impact on dough rheology and the quality of bread possibly due to effects on the gluten network or on gliadin.
Proteases are also applied in the manufacture of pastries, biscuits, and cookies. They act on the proteins of wheat flour, reducing gluten elasticity and therefore reducing shrinkage of dough or paste after molding and sheeting; for instance, hydrolysis of glutenin proteins, which are responsible for the elasticity of dough, has considerable improving effects on the spread ratio of cookies.
Hemicellulases
Hemicellulases are a diverse class of enzymes that hydrolyse hemicelluloses, a group of pol‐ ysaccharides comprising xylan, xylobiose, arabinoxylan, and arabinogalactan. This group includes xylanase or endo-1,4-β-xylanase (4-β-D-xylan xylanohydrolase), a glycosidase that catalyses the endohydrolysis of 1,4-β-D-xylosidic linkages in xylan and ara‐ binoxylan. Xylanase, also designated endoxylanase, was originally termed pentosanase. A wide variety of xylanases have been reported from a plethora of microorganisms including bacteria, archaea, and fungi. These enzymes are mainly classified in the glycosyl hydrolase (GH) families 10 and 11, although putative xylanase activities have been reported in GH families 5, 7, 8 and 43. GH10 xylanases are regarded to have broader substrate specificity and release shorter fragments compared to GH11 xylanases, while the latter enzymes are more susceptible to steric hindrance by arabinose substituents.
Xylanases were introduced to the baking segment in the years 1970 and are most often used combined with amylases, lipases, and many oxidoreductases to attain specific effects on the rheological properties of dough and organoleptic properties of bread. These enzymes have also been used to improve the quality of biscuits, cakes and other baked products. The most favorable xylanases for bread-making are those that preferentially act on WU-AX and are poorly active on WE-AX, because they remove the insoluble arabinoxylans which interfere with the formation of the gluten network, giving rise to high molecular weight solubilised arabinoxylans, resulting in increased viscosity and thus enhancing dough stability.
As a consequence, a more stable, flexible and easy to handle dough is obtained, resulting in improved oven spring, larger loaf volume, as well as a softer crumb with improved structure. Moreover, the addition of xylanases during dough processing is expected to increase the concentration of arabinoxylo-oligosaccharides in bread, which have beneficial effects on human health.
Although both enzymes had a positive effect on loaf volume, psychrophilic GH8 xylanase was apparently much more efficient than the mesophilic enzyme from the same family, because much lower concentrations of the former enzyme were required to produce a similar increase in bread volume.
Lipases
Lipases or triacylglycerol acylhydrolases hydrolyse triacylglycerols (TAG) producing monoacylglycerols (MAG), diacylglycerols (DAG), glycerol and free fatty acids. These enzymes are widely found in nature. Besides TAG lipases there are phospholipases A1, A2, C, D, and galactolipases. Even though they are present in all cereal grains; lipase activity of white flour is usually low enough to avoid rancidity due to hydrolysis of native lipids and of baking fat. The use of lipases in the baking segment is much more recent in comparison to α-amylases and proteases.
The first generation of commercial lipase preparations was introduced to the market in the years 1990 and recently a third generation became available. The latter are protein engineered enzymes, claimed to give a better effect in high-speed mixing and no time dough processes. Moreover, third generation lipases have a lower affinity for short-chain fatty acids, which reduces the risk for off-flavour formation on account of prolonged storage of the baked goods and the use of butter or milk fat in baked products. Lipases (TAG lipases) of the first generation are 1,3-specific, removing preferentially fatty acids from positions 1 and 3 in TAG. These enzymes can improve dough rheology, increase dough strength and stability, thus improving dough machinability. In addition, lipases lead to an increase in volume which results in an improved, more uniform crumb structure; hence a softer crumb is obtained.
The second-generation lipases act simultaneously on TAG, diacylgalactolipids and phospholipids, producing more polar lipids, providing a greater increase in volume, better stability to mechanical stress on the dough, and a fine, uniform bread crumb structure compared to the first generation lipases. Moreover, a third-generation lipase was found to increase expansion of the gluten network, increase the wall thickness and reduce cell density, enhancing volume and crumb structure of high fibre white bread.
In this context, the roles of lipids and surfactants in bread-making have been extensively reviewed elsewhere. The addition of lipases has been claimed to retard the rate of staling in baked products. The effect of these enzymes has been attributed to in situ production of MAG following TAG hydrolysis, although this mechanism is not completely accepted because the amount of MAG would be insufficient to account for the antistaling effect.
Lipases may also be used for the development of particular flavors in bakery products. The effect of a third generation lipase on the quality of high-fiber enriched brewer’s spent grain bread has been evaluated. The enzyme produced beneficial effects during bread making, positively affecting loaf volume, staling rate, and crumb structure.
The application of lipase and MAG to produce fibre enriched pan bread using the straight dough method was assessed. The use of lipase dosages up to 50 ppm and MAG up to 2% indicated the possibility of replacement of MAG by lipases in fibre enriched pan bread. Recently, the effects of two lipases and DATEM on the rheological and thermal properties of white and whole wheat flour doughs were compared.
Lipases were able to cause modifications in the dough components (gluten proteins and starch). The enzymes improved dough handling properties to a similar or greater extent than DATEM, increasing dough stability, maximum resistance to extension and hardness, and decreasing softening degree and stickiness. The possible role of lipases in delaying starch retrogradation was indicated by the greater extent of formation of amylose-lipid complexes promoted by lipases in comparison to DATEM.
FUNGAL ALPHA AMYLASE
Food grade alpha amylase from a fungal origin used specifically in the baking industry.

Enhances Fermentation
Process

Improves Bread Volume

Improves Crumb Texture
LIPASE
Lipase enzymes for the baking industry can replace chemical based dough improvers.

Increases Dough Tolerance

Improves Loaf Volume

Decreased Stickiness
OTHER BAKERY ENZYMES
We also produce different Bakery Enzymes for various applications.

Improves Baking Quality

Stronger Dough

Reduce Microbial Spoilage
Related Articles
Enzymes Used as Protein Binders in Dairy Products
In the dairy industry, enzymes play a crucial role in the production of various products. One of the applications of enzymes is their use as protein binders in dairy products. Enzymes are natural proteins that act as catalysts, speeding up chemical reactions within a...
Enzymes in the Baking Industry: 10 Dough Conditioning Solutions
Enzymes play a crucial role in the baking industry, offering many benefits that improve dough consistency, product quality, and production efficiency. Understanding the science behind enzymes and their functions is essential for bakers looking to enhance their baking...
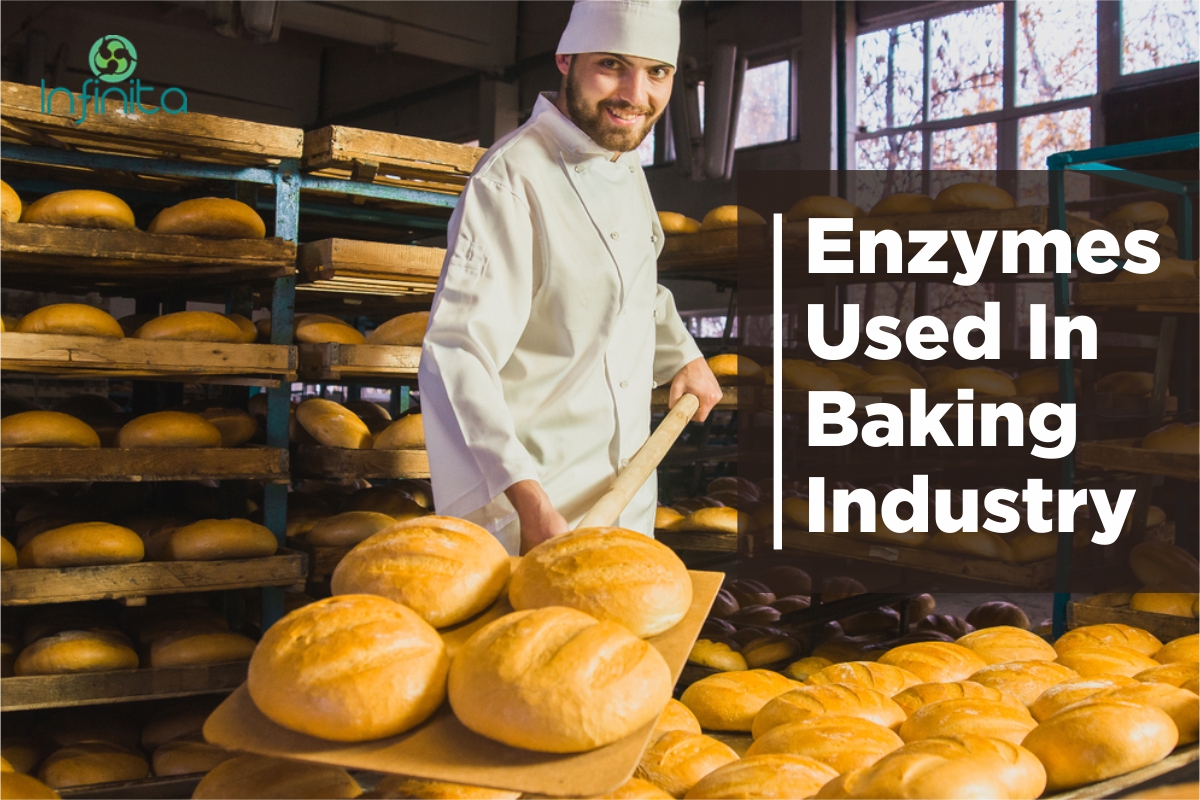
Enzymes Used In Baking Industry
Enzymes Used In Baking Industry
The use of enzymes dates from much longer than their ability to catalyse reactions was recognised and their chemical nature was known. The first completely enzymatic industrial process was developed in the years 1960. Starch processing, which is undertaken in two steps, involves liquefaction of the polysaccharide using bacterial α-amylase, followed by saccharification catalysed by fungal glucoamylase.
Developments in process technology allied to the use of recombinant techniques during the last decades allowed for considerably improved yields by fermentation increased stability and altered specificity and selectivity of enzymes. Those techniques thrust forward and are continuing to broaden the applications of enzymes in food technology and many different areas.
There are two scenarios regarding the use of enzymes, either the enzymes are used to convert the raw material into the main product, or the enzymes are used as additives to alter a functional characteristic of the product. In the first case, the enzymatic process is undertaken in optimised and controlled conditions to enhance the catalytic potential of the enzyme, whereas in the second situation it is more difficult to assure optimal conditions and to control the enzymatic reaction.
Bakery Enzymes Industry
The development of the bread process was an important event in mankind. An important aspect that contributed to the evolution of the baking market was the introduction of industrial enzymes in the baking process, where bakery enzymes represent a relevant segment of the industry.
Main Constituents Of Baked-Products
Baking is a common name for the production of baked goods, such as bread, cake, pastries, biscuits, crackers, cookies, pies and tortillas, where wheat flour is both the most essential ingredient and a key source of enzyme substrates for the product. Even though based on cereals other than wheat, baked goods such as gluten-free products or rye bread are also considered to be baked products.
Bread is usually made from wheat flour as raw material, which is a mixture of starch, gluten, lipids, non-starch polysaccharides, and enzymes. After flour, yeast, and water are mixed, complex biochemical and biophysical processes begin, catalysed by the wheat enzymes and by the yeast, characterising the dough phase. These processes go on in the baking phase, giving rise to bread.
Extra enzymes added to the dough improve control of the baking process, allowing the use of different baking processes, reducing process time, slowing-down staling, compensating for flour variability and substituting chemical additives.
Starch is the main component of products such as bread and other bakery goods and is added to different foods, acting as a thickener, water binder, emulsion stabiliser, gelling agent and fat substitute. It is the most abundant constituent and most important reserve polysaccharide of many plants, including cereals, occurring as intracellular, semi-crystalline granules. On a molecular level, its major components are the glucose polymers amylose and amylopectin.
Amylose is an essentially linear molecule, consisting of up to 6000 glucose units with α-(1,4)-glycosidic bonds. On the other hand, amylopectin is a highly branched polysaccharide constituted of short α-1,4 linked linear chains of 10–60 glucose units and α-1,6 linked side chains with 15–45 glucose units, containing on average 2 million glucose units.
Enzymes Used In Baked Products
Baking comprises the use of enzymes from three sources: the endogenous enzymes in flour, enzymes associated with the metabolic activity of the dominant microorganisms and exogenous enzymes which are added in the dough. The supplementation of flour and dough with enzyme improvers is a usual practice for flour standardisation and also as baking aids.
Enzymes are usually added to modify dough rheology, gas retention and crumb softness in bread manufacture, to modify dough rheology in the manufacture of pastry and biscuits, to change product softness in cake making and to reduce acrylamide formation in bakery products. The enzymes can be added individually or in complex mixtures, which may act in a synergistic way in the production of baked goods [60-62], and their levels are usually very low.
Hydrolases
Enzymes as technological aids are usually added to flour, during the mixing step of the bread-making process. The enzymes most frequently used in bread-making are the α-amylases from different origins. Amylases and other starch-converting enzymes The industrial processing of starch is usually started by α-amylases (α-1,4-glucanohydro‐ lase). Most of the starch-converting enzymes belong to the α-amylase family or family 13 glycosyl hydrolases (GH), based on amino acid se α-Amylases are endo-enzymes that catalyse the cleavage of α-1,4-glycosidic bonds in the inner part of the amylose or amylopectin chain. The end products of α-amylase action are oligosaccharides, with an α-configuration and varying lengths, and α-limit dextrins, which are branched oligosaccharides. These enzymes can be obtained from cereal, fungal, bacterial and biotechnologically altered bacterial sources.
Differences in the number of binding sites and location of catalytic regions determine the substrate specificity of α-amylases, the length of the oligosaccharide fragments released after hydrolysis and, consequently, the carbohydrate profile of the final product. The increased levels of reducing sugars lead to the formation of Maillard reaction products, intensifying bread flavour and crust colour. In addition, these enzymes can improve the gas-retention properties of fermented dough and reduce dough viscosity during starch gelatinisation, with consequent improvements in product volume and softness. Certain amylases are able to decrease the firming rate of bread crumb, acting as anti-staling agents.
Amylase-containing anti-staling products typically consist of bacterial or fungal α296 Food Industry amylases with intermediate thermostability. In this context, one of the most effective anti-staling amylases is the Bacillus stearothermophilus maltogenic α-amylase. The anti-staling action of amylases has been attributed to the modified retrogradation behavior of the hydro‐ lysed starch. Yet, other researchers describe the effect of the interference of the low molecular weight dextrins with starch-starch and/or gluten-starch interactions.
Proteases
Proteases can be subdivided into two major groups according to their site of action: exopeptidases and endopeptidases. Exopeptidases cleave the peptide bond proximal to the amino or carboxy termini of the substrate, whereas endopeptidases cleave peptide bonds distant from the termini of the substrate. Most of the proteolytic activity of wheat and rye flours corresponds to aspartic proteases and carboxypeptidases, which are both active in acid pH. Additionally, aspartic proteases of wheat are partly associated with gluten. Nevertheless, the proteolytic activity of sound, ungerminated grain is normally low.
Proteases are used on a large commercial scale in the production of bread, baked goods, crackers, and waffles. These enzymes can be added to reduce mixing time, to decrease dough consistency, to assure dough uniformity, to regulate gluten strength in bread, to control bread texture and to improve flavor. In addition, proteases have largely replaced bi-sulfite, which was previously used to control consistency through reduction of gluten protein disulfide bonds, while proteolysis breaks down peptide bonds. In both cases, the final effect is a similar weakening of the gluten network. In bread production, a fungal acid protease is used to modify mixtures containing high gluten content. When proteases are mixed in the blend, it undergoes partial hydrolysis becoming soft and easy to pull and knead. Proteases are also frequently added to dough preparations. These enzymes have a great impact on dough rheology and the quality of bread possibly due to effects on the gluten network or on gliadin.
Proteases are also applied in the manufacture of pastries, biscuits, and cookies. They act on the proteins of wheat flour, reducing gluten elasticity and therefore reducing shrinkage of dough or paste after molding and sheeting; for instance, hydrolysis of glutenin proteins, which are responsible for the elasticity of dough, has considerable improving effects on the spread ratio of cookies.
Hemicellulases
Hemicellulases are a diverse class of enzymes that hydrolyse hemicelluloses, a group of pol‐ ysaccharides comprising xylan, xylobiose, arabinoxylan, and arabinogalactan. This group includes xylanase or endo-1,4-β-xylanase (4-β-D-xylan xylanohydrolase), a glycosidase that catalyses the endohydrolysis of 1,4-β-D-xylosidic linkages in xylan and ara‐ binoxylan. Xylanase, also designated endoxylanase, was originally termed pentosanase. A wide variety of xylanases have been reported from a plethora of microorganisms including bacteria, archaea, and fungi. These enzymes are mainly classified in the glycosyl hydrolase (GH) families 10 and 11, although putative xylanase activities have been reported in GH families 5, 7, 8 and 43. GH10 xylanases are regarded to have broader substrate specificity and release shorter fragments compared to GH11 xylanases, while the latter enzymes are more susceptible to steric hindrance by arabinose substituents.
Xylanases were introduced to the baking segment in the years 1970 and are most often used combined with amylases, lipases, and many oxidoreductases to attain specific effects on the rheological properties of dough and organoleptic properties of bread. These enzymes have also been used to improve the quality of biscuits, cakes and other baked products. The most favorable xylanases for bread-making are those that preferentially act on WU-AX and are poorly active on WE-AX, because they remove the insoluble arabinoxylans which interfere with the formation of the gluten network, giving rise to high molecular weight solubilised arabinoxylans, resulting in increased viscosity and thus enhancing dough stability.
As a consequence, a more stable, flexible and easy to handle dough is obtained, resulting in improved oven spring, larger loaf volume, as well as a softer crumb with improved structure. Moreover, the addition of xylanases during dough processing is expected to increase the concentration of arabinoxylo-oligosaccharides in bread, which have beneficial effects on human health.
Although both enzymes had a positive effect on loaf volume, psychrophilic GH8 xylanase was apparently much more efficient than the mesophilic enzyme from the same family, because much lower concentrations of the former enzyme were required to produce a similar increase in bread volume.
Lipases
Lipases or triacylglycerol acylhydrolases hydrolyse triacylglycerols (TAG) producing monoacylglycerols (MAG), diacylglycerols (DAG), glycerol and free fatty acids. These enzymes are widely found in nature. Besides TAG lipases there are phospholipases A1, A2, C, D, and galactolipases. Even though they are present in all cereal grains; lipase activity of white flour is usually low enough to avoid rancidity due to hydrolysis of native lipids and of baking fat. The use of lipases in the baking segment is much more recent in comparison to α-amylases and proteases.
The first generation of commercial lipase preparations was introduced to the market in the years 1990 and recently a third generation became available. The latter are protein engineered enzymes, claimed to give a better effect in high-speed mixing and no time dough processes. Moreover, third generation lipases have a lower affinity for short-chain fatty acids, which reduces the risk for off-flavour formation on account of prolonged storage of the baked goods and the use of butter or milk fat in baked products. Lipases (TAG lipases) of the first generation are 1,3-specific, removing preferentially fatty acids from positions 1 and 3 in TAG. These enzymes can improve dough rheology, increase dough strength and stability, thus improving dough machinability. In addition, lipases lead to an increase in volume which results in an improved, more uniform crumb structure; hence a softer crumb is obtained.
The second-generation lipases act simultaneously on TAG, diacylgalactolipids and phospholipids, producing more polar lipids, providing a greater increase in volume, better stability to mechanical stress on the dough, and a fine, uniform bread crumb structure compared to the first generation lipases. Moreover, a third-generation lipase was found to increase expansion of the gluten network, increase the wall thickness and reduce cell density, enhancing volume and crumb structure of high fibre white bread.
In this context, the roles of lipids and surfactants in bread-making have been extensively reviewed elsewhere. The addition of lipases has been claimed to retard the rate of staling in baked products. The effect of these enzymes has been attributed to in situ production of MAG following TAG hydrolysis, although this mechanism is not completely accepted because the amount of MAG would be insufficient to account for the antistaling effect.
Lipases may also be used for the development of particular flavors in bakery products. The effect of a third generation lipase on the quality of high-fiber enriched brewer’s spent grain bread has been evaluated. The enzyme produced beneficial effects during bread making, positively affecting loaf volume, staling rate, and crumb structure.
The application of lipase and MAG to produce fibre enriched pan bread using the straight dough method was assessed. The use of lipase dosages up to 50 ppm and MAG up to 2% indicated the possibility of replacement of MAG by lipases in fibre enriched pan bread. Recently, the effects of two lipases and DATEM on the rheological and thermal properties of white and whole wheat flour doughs were compared.
Lipases were able to cause modifications in the dough components (gluten proteins and starch). The enzymes improved dough handling properties to a similar or greater extent than DATEM, increasing dough stability, maximum resistance to extension and hardness, and decreasing softening degree and stickiness. The possible role of lipases in delaying starch retrogradation was indicated by the greater extent of formation of amylose-lipid complexes promoted by lipases in comparison to DATEM.
0 Comments
Submit a Comment
You must be logged in to post a comment.
FUNGAL ALPHA AMYLASE
Food grade alpha amylase from a fungal origin used specifically in the baking industry.

Enhances Fermentation Process

Improves Bread Volume

Improves Crumb Texture
LIPASE
Lipase enzymes for the baking industry can replace chemical based dough improvers.

Increases Dough Tolerance

Improves Loaf Volume

Decreased Stickiness
OTHER BAKERY ENZYMES
We also produce different Bakery Enzymes for various applications.

Improves Baking Quality

Stronger Dough

Reduce Microbial Spoilage
Enzymes Used as Protein Binders in Dairy Products
In the dairy industry, enzymes play a crucial role in the production of various products. One of the applications of enzymes is their use as protein binders in dairy products. Enzymes are natural proteins that act as catalysts, speeding up chemical reactions within a...
Enzymes in the Baking Industry: 10 Dough Conditioning Solutions
Enzymes play a crucial role in the baking industry, offering many benefits that improve dough consistency, product quality, and production efficiency. Understanding the science behind enzymes and their functions is essential for bakers looking to enhance their baking...
5 Ways Dairy Enzymes Are Revolutionizing the Food Industry
Dairy enzymes are making a significant impact on the food industry, transforming the way we produce and consume dairy products. These powerful enzymes play a crucial role in various stages of food manufacturing, from enhancing food quality and shelf life to reducing...
0 Comments