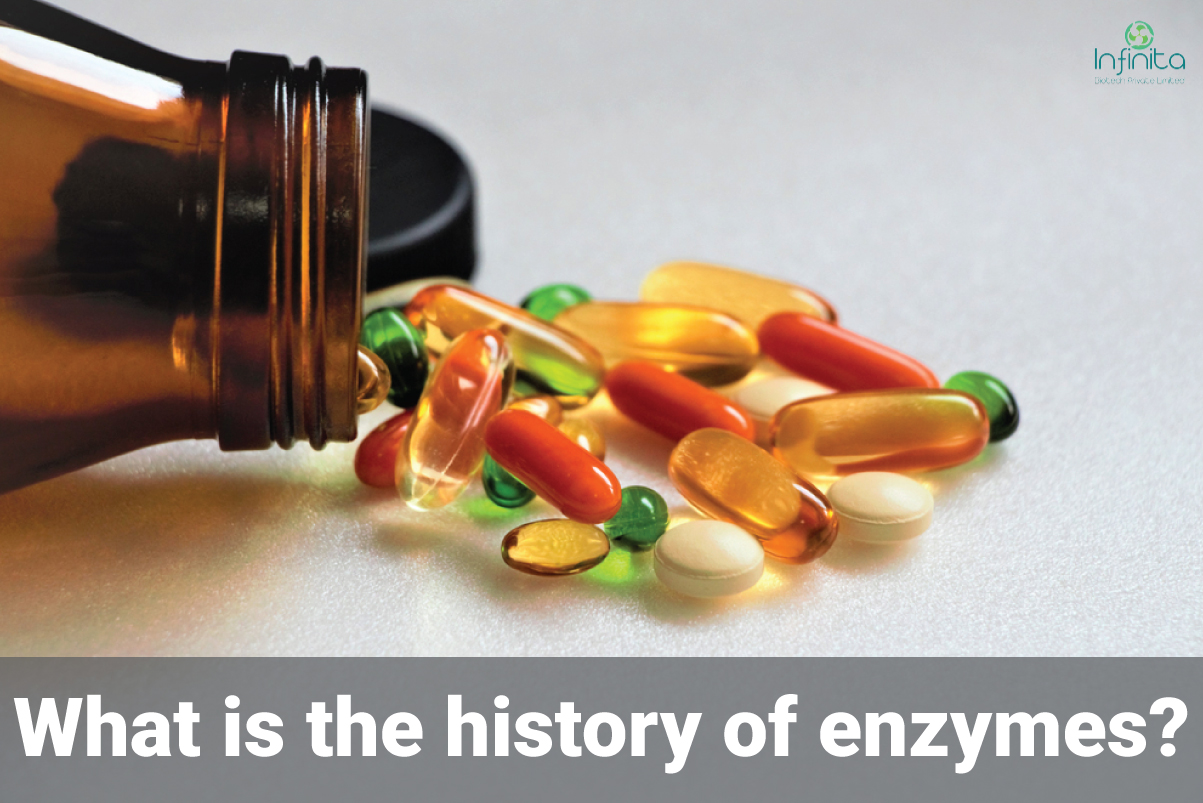
History Of Enzymes
What Is The History Of Enzymes?
Enzymes are macromolecular biological catalysts. Enzymes accelerate chemical reactions. The molecules upon that proteins might act are referred to as substrates and therefore the enzyme converts the substrates into totally different molecules referred to as merchandise. Almost all metabolic processes within the cell would like protein chemical process so as to occur at rates quickly enough to sustain life. Metabolic pathways rely on enzymes to turn individual steps. The study of enzymes is called enzymology and a new field of pseudo enzyme analysis has recently grown up, recognising that during evolution, some enzymes have lost the ability to carry out the biological chemical process, which is often reflected in their amino acid sequences and unusual ‘pseudo catalytic’ properties.
History of Enzymes states that Enzymes are best-known to turn quite five,000 biochemical reaction types. Most enzymes are proteins, although a few are catalytic RNA molecules. The latter are called ribozymes. Enzymes’ specificity comes from their unique three-dimensional structures.
Some enzymes will build their conversion of substrate to product occur several lots of times quicker. An extreme example is orotidine 5′-phosphate enzyme, which allows a reaction that would otherwise take millions of years to occur in milliseconds. Chemically, enzymes are like every catalyst and aren’t consumed in chemical reactions, nor do they alter the equilibrium of a reaction. Enzymes take issue from most alternative catalysts by being rather more specific. Enzyme activity will be suffering from alternative molecules: inhibitors are molecules that decrease protein activity, and activators are molecules that increase activity. Many therapeutic drugs and poisons are enzyme inhibitors. An enzyme’s activity decreases markedly outside its optimal temperature and pH, and many enzymes are (permanently) denatured when exposed to excessive heat, losing their structure and catalytic properties.
Some enzymes are used commercially, as an example, in the synthesis of antibiotics. Some house merchandise use enzymes to hurry up chemical reactions: enzymes in biological laundry powders break down a super molecule, starch or fat stains on garments.
French chemist Anselme Payen was the primary to get Associate in Nursing protein, diastase, in 1833. A few decades later, once learning the fermentation of sugar to alcohol by yeast, Louis Pasteur concluded that this fermentation was caused by a vital force contained within the yeast cells referred to as “ferments”, that were thought to operate solely at intervals living organisms.
In 1877, German life scientist Wilhelm Kühne (1837–1900) initially used the term protein, which comes from Greek, “leavened” or “in yeast”, to describe this process. The word protein was used later to talk to dead substances like enzyme, and therefore the word ferment was accustomed talk to chemical activity created by living organisms.
Eduard Buchner submitted his initial paper on the study of yeast extracts in 1897. In a series of experiments at the University of Berlin, he found that sugar was fermented by yeast extracts even when there were no living yeast cells in the mixture. He named the protein that led to the fermentation of disaccharide “zymase”. In 1907, he received the honour in Chemistry for “his discovery of non-cellular fermentation” is the evidence of the history of enzymes.
The organic chemistry identity of enzymes was still unknown within the early decennium. Many scientists determined that protein activity was related to proteins, however others (such as Nobel Prize Winner Richard Willstätter) argued that proteins were simply carriers for actuality enzymes and that proteins per se were incapable of catalysis. In 1926, James B. Sumner showed that the catalyst enzyme was a pure macromolecule and crystallized it; he did likewise for the catalyst enzyme in 1937. The conclusion that pure proteins will be enzymes was definitively incontestable by John Howard biochemist and Wendell Meredith Stanley, who worked on the digestive enzymes pepsin (1930), trypsin and chymotrypsin. These 3 scientists were awarded the 1946 laurels in Chemistry.
The history of enzymes discovers that enzymes can be crystallised eventually allowed their structures to be resolved by x-ray natural philosophy. This was initially in deep trouble enzyme, an enzyme found in tears, saliva and egg whites that digests the coating of some bacteria; the structure was solved by a group led by David Chilton Phillips and published in 1965. This high-resolution structure of lysozyme marked the beginning of the field of structural biology and the effort to understand how enzymes work at an atomic level of detail.
Naming Conventions
An enzyme’s name is usually derived from its substrate or the chemical change it catalyses, with the word ending in -ase. Examples are lactase, alcohol dehydrogenase, and DNA polymerase. Different enzymes that catalyse identical chemical change are referred to as isozymes.
The International Union of chemical science and biology have developed a language for enzymes, the EC numbers; every accelerator is represented by a sequence of 4 numbers preceded by “EC”, which stands for “Enzyme Commission”. The first variety broadly speaking classifies the catalyst supported its mechanism.
The top-level classification is:
EC 1, Oxidoreductases: catalyse oxidation/reduction reactions
EC 2, Transferases: transfer a functional group (e.g. a methyl or phosphate group)
EC 3, Hydrolases: catalyse the reaction of assorted bonds
EC 4, Lyases: cleave numerous bonds by means that aside from reaction and chemical reaction
EC 5, Isomerases: catalyse isomerisation changes inside one molecule
EC 6, Ligases: be a part of 2 molecules with valence bonds.
These sections are divided by different options like the substrate, products, and chemical change. An enzyme is fully specified by four numerical designations. For example, hexokinase (EC 2.7.1.1) is a transferase (EC 2) that adds a phosphate group (EC 2.7) to a hexose sugar, a molecule containing an alcohol group (EC 2.7.1).
Structure
Enzymes are usually spherical proteins, acting alone or in larger complexes. The sequence of the amino acids specifies the structure that successively determines the chemical process activity of the catalyst. Although structure determines to operate, unique protein activity cannot nevertheless be foreseen from structure alone. Enzyme structures unfold (denature) once heated or exposed to chemical denaturants and this disruption to the structure generally causes a loss of activity. Enzyme denaturation traditionally is generally being often} coupled to temperatures on top of a species’ normal level; as a result, enzymes from bacteria living in volcanic environments such as hot springs are prized by industrial users for his or her ability to operate at high temperatures, allowing enzyme-catalysed reactions to be operated at a very high rate.
Enzymes are usually much larger than their substrates. Sizes vary from simply sixty-two aminoalkanoic acid residues, for the monomer of 4-oxalocrotonate tautomerase, to over 2,500 residues in the animal fatty acid synthase. Only a tiny low portion of their structure (around 2–4 amino acids) is directly concerned in catalysis: the chemical process website. This catalytic site is located next to one or more binding sites where residues orient the substrates. The chemical process {site} and binding site along comprise the enzyme’s site. The remaining majority of the catalyst structure serves to keep up the precise orientation and dynamics of the site.
In some enzymes, no amino acids are directly concerned in catalysis; instead, the enzyme contains sites to bind and orient catalytic cofactors. Enzyme structures may additionally contain allosteric sites wherever the binding of a tiny low molecule causes a conformational amendment that will increase or decreases activity.
A small variety of RNA-based biological catalysts referred to as ribozymes exist, which again can act alone or in complex with proteins. The most common of these is the ribosome which is a complex of protein and catalytic RNA components.
Mechanism
Substrate Binding
Enzymes should bind their substrates before they’ll catalyse any chemical change. Enzymes are usually very specific as to what substrates they bind and then the chemical reaction catalysed. Specificity is achieved by binding pockets with complementary form, charge and hydrophilic/hydrophobic characteristics to the substrates. Enzymes will so distinguish between terribly similar substrate molecules to be chemoselective, regioselective and stereospecific.
Some of the enzymes showing the best specificity and accuracy are concerned within the repetition and expression of the order. Some of these enzymes have “proof-reading” mechanisms. Here, an associate catalyst like DNA enzyme catalyses a reaction in an exceedingly beginning and so checks that the merchandise is correct in an exceedingly second step. This ballroom dance method leads to average error rates of but one error in one hundred million reactions in hi-fi class polymerases. Similar proofreading mechanisms also are found in polymer enzyme, aminoacyl tRNA synthetases, and ribosomes.
Conversely, some catalysts show enzyme sexual activity, having broad specificity and acting on a range of different physiologically relevant substrates. Many enzymes possess tiny aspect activities that arose luckily (i.e. neutrally), which may be the starting point for the evolutionary selection of a new function.
“Lock And Key” Model
To explain the discovered specificity of enzymes, in 1894 Emil Fischer projected that each the protein and also the substrate possess specific complementary geometric shapes that match precisely into each other. This is typically said as “the lock and key” model. This early model explains protein specificity but fails to explain the stabilization of the transition state that enzymes achieve.
“Induced Fit” Model
In 1958, Daniel Koshland recommended a modification to the lock and key model: since enzymes square measure rather versatile structures, the active site is continuously reshaped by interactions with the substrate as the substrate interacts with the enzyme. As a result, the substrate does not simply bind to a rigid active site; the amino acid side-chains that make up the active site are moulded into the precise positions that alter the protein to perform its chemical process perform. In some cases, such as glycosidases, the substrate molecule also changes shape slightly as it enters the active site. The situation continues to vary until the substrate is totally sure, at which point the final shape and charge distribution is determined.
Catalysis
Enzymes will accelerate reactions in many ways in which, all of which lower the activation energy (ΔG‡, Gibbs free energy)
- By stabilizing the transition state:
Creating AN surroundings with a charge distribution complementary to it of the transition state to lower its energy
- By providing an alternative reaction pathway:
Temporarily reacting with the substrate, forming a valence intermediate to supply a lower energy transition state
- By destabilising the substrate ground state:
Distorting bound substrate(s) into their transition state form to reduce the energy required to reach the transition state
By oriented the substrates into a productive arrangement to cut back the reaction entropy amendment (the contribution of this mechanism to chemical action is comparatively small)
Enzymes may use several of these mechanisms simultaneously. For example, proteases such as trypsin perform covalent catalysis using a catalytic triad, stabilise charge build-up on the transition states using an oxyanion hole, complete hydrolysis using an oriented water substrate.
Dynamics
Enzymes don’t seem to be rigid, static structures; instead they have complex internal dynamic motions – that is, movements of parts of the enzyme’s structure such as individual amino acid residues, groups of residues forming a macromolecule loop or unit of secondary structure, or even an entire protein domain. These motions make to a conformational ensemble of slightly completely different structures that interconvert with each other at equilibrium. Different states inside this ensemble could also be related to completely different aspects of AN enzymes perform. For example, completely different conformations of the protein dihydrofolate enzyme square measure related to the substrate binding, catalysis, cofactor release, and product release steps of the catalytic cycle.
Allosteric Modulation
Allosteric sites square measure pockets on the protein, distinct from the active site, that bind to molecules in the cellular environment. These molecules then cause a change in the conformation or dynamics of the enzyme that is transduced to the active site and thus affects the reaction rate of the enzyme. In this way, allosteric interactions can either inhibit or activate enzymes. Allosteric interactions with metabolites upstream or downstream in AN enzyme’s metabolic pathway cause feedback regulation, fixing the activity of the protein in step with the flux through the remainder of the pathway.
Related Articles
The Basics of Soil Stabilization: An Introduction
What is Soil Stabilization? Definition: Have you ever wondered how builders manage to create rock-solid foundations for structures, even on unstable grounds? That's where soil stabilization becomes critical. At its core, soil stabilization refers to the process of...
Bio Enzyme Uses and Their Applications
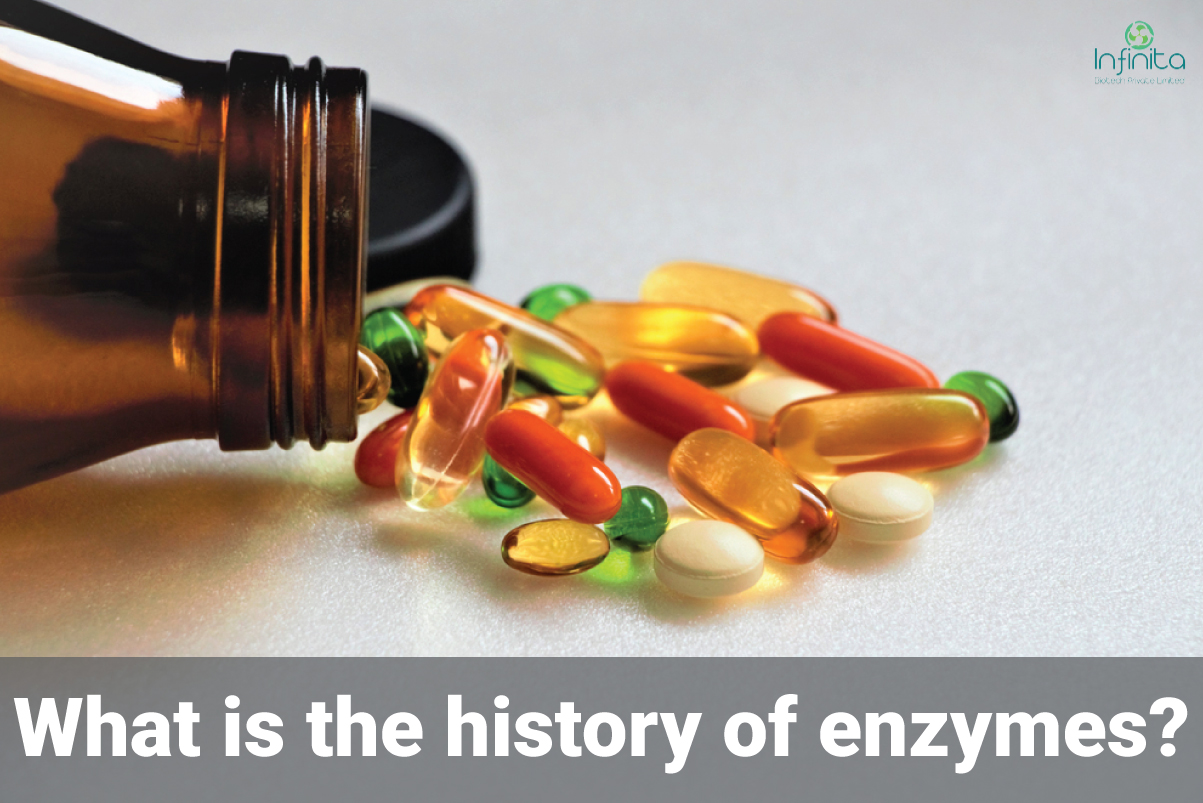
History Of Enzymes
What Is The History Of Enzymes?
Enzymes are macromolecular biological catalysts. Enzymes accelerate chemical reactions. The molecules upon that proteins might act are referred to as substrates and therefore the enzyme converts the substrates into totally different molecules referred to as merchandise. Almost all metabolic processes within the cell would like protein chemical process so as to occur at rates quickly enough to sustain life. Metabolic pathways rely on enzymes to turn individual steps. The study of enzymes is called enzymology and a new field of pseudo enzyme analysis has recently grown up, recognising that during evolution, some enzymes have lost the ability to carry out the biological chemical process, which is often reflected in their amino acid sequences and unusual ‘pseudo catalytic’ properties.
History of Enzymes states that Enzymes are best-known to turn quite five,000 biochemical reaction types. Most enzymes are proteins, although a few are catalytic RNA molecules. The latter are called ribozymes. Enzymes’ specificity comes from their unique three-dimensional structures.
Some enzymes will build their conversion of substrate to product occur several lots of times quicker. An extreme example is orotidine 5′-phosphate enzyme, which allows a reaction that would otherwise take millions of years to occur in milliseconds. Chemically, enzymes are like every catalyst and aren’t consumed in chemical reactions, nor do they alter the equilibrium of a reaction. Enzymes take issue from most alternative catalysts by being rather more specific. Enzyme activity will be suffering from alternative molecules: inhibitors are molecules that decrease protein activity, and activators are molecules that increase activity. Many therapeutic drugs and poisons are enzyme inhibitors. An enzyme’s activity decreases markedly outside its optimal temperature and pH, and many enzymes are (permanently) denatured when exposed to excessive heat, losing their structure and catalytic properties.
Some enzymes are used commercially, as an example, in the synthesis of antibiotics. Some house merchandise use enzymes to hurry up chemical reactions: enzymes in biological laundry powders break down a super molecule, starch or fat stains on garments.
French chemist Anselme Payen was the primary to get Associate in Nursing protein, diastase, in 1833. A few decades later, once learning the fermentation of sugar to alcohol by yeast, Louis Pasteur concluded that this fermentation was caused by a vital force contained within the yeast cells referred to as “ferments”, that were thought to operate solely at intervals living organisms.
In 1877, German life scientist Wilhelm Kühne (1837–1900) initially used the term protein, which comes from Greek, “leavened” or “in yeast”, to describe this process. The word protein was used later to talk to dead substances like enzyme, and therefore the word ferment was accustomed talk to chemical activity created by living organisms.
Eduard Buchner submitted his initial paper on the study of yeast extracts in 1897. In a series of experiments at the University of Berlin, he found that sugar was fermented by yeast extracts even when there were no living yeast cells in the mixture. He named the protein that led to the fermentation of disaccharide “zymase”. In 1907, he received the honour in Chemistry for “his discovery of non-cellular fermentation” is the evidence of the history of enzymes.
The organic chemistry identity of enzymes was still unknown within the early decennium. Many scientists determined that protein activity was related to proteins, however others (such as Nobel Prize Winner Richard Willstätter) argued that proteins were simply carriers for actuality enzymes and that proteins per se were incapable of catalysis. In 1926, James B. Sumner showed that the catalyst enzyme was a pure macromolecule and crystallized it; he did likewise for the catalyst enzyme in 1937. The conclusion that pure proteins will be enzymes was definitively incontestable by John Howard biochemist and Wendell Meredith Stanley, who worked on the digestive enzymes pepsin (1930), trypsin and chymotrypsin. These 3 scientists were awarded the 1946 laurels in Chemistry.
The history of enzymes discovers that enzymes can be crystallised eventually allowed their structures to be resolved by x-ray natural philosophy. This was initially in deep trouble enzyme, an enzyme found in tears, saliva and egg whites that digests the coating of some bacteria; the structure was solved by a group led by David Chilton Phillips and published in 1965. This high-resolution structure of lysozyme marked the beginning of the field of structural biology and the effort to understand how enzymes work at an atomic level of detail.
Naming Conventions
An enzyme’s name is usually derived from its substrate or the chemical change it catalyses, with the word ending in -ase. Examples are lactase, alcohol dehydrogenase, and DNA polymerase. Different enzymes that catalyse identical chemical change are referred to as isozymes.
The International Union of chemical science and biology have developed a language for enzymes, the EC numbers; every accelerator is represented by a sequence of 4 numbers preceded by “EC”, which stands for “Enzyme Commission”. The first variety broadly speaking classifies the catalyst supported its mechanism.
The top-level classification is:
EC 1, Oxidoreductases: catalyse oxidation/reduction reactions
EC 2, Transferases: transfer a functional group (e.g. a methyl or phosphate group)
EC 3, Hydrolases: catalyse the reaction of assorted bonds
EC 4, Lyases: cleave numerous bonds by means that aside from reaction and chemical reaction
EC 5, Isomerases: catalyse isomerisation changes inside one molecule
EC 6, Ligases: be a part of 2 molecules with valence bonds.
These sections are divided by different options like the substrate, products, and chemical change. An enzyme is fully specified by four numerical designations. For example, hexokinase (EC 2.7.1.1) is a transferase (EC 2) that adds a phosphate group (EC 2.7) to a hexose sugar, a molecule containing an alcohol group (EC 2.7.1).
Structure
Enzymes are usually spherical proteins, acting alone or in larger complexes. The sequence of the amino acids specifies the structure that successively determines the chemical process activity of the catalyst. Although structure determines to operate, unique protein activity cannot nevertheless be foreseen from structure alone. Enzyme structures unfold (denature) once heated or exposed to chemical denaturants and this disruption to the structure generally causes a loss of activity. Enzyme denaturation traditionally is generally being often} coupled to temperatures on top of a species’ normal level; as a result, enzymes from bacteria living in volcanic environments such as hot springs are prized by industrial users for his or her ability to operate at high temperatures, allowing enzyme-catalysed reactions to be operated at a very high rate.
Enzymes are usually much larger than their substrates. Sizes vary from simply sixty-two aminoalkanoic acid residues, for the monomer of 4-oxalocrotonate tautomerase, to over 2,500 residues in the animal fatty acid synthase. Only a tiny low portion of their structure (around 2–4 amino acids) is directly concerned in catalysis: the chemical process website. This catalytic site is located next to one or more binding sites where residues orient the substrates. The chemical process {site} and binding site along comprise the enzyme’s site. The remaining majority of the catalyst structure serves to keep up the precise orientation and dynamics of the site.
In some enzymes, no amino acids are directly concerned in catalysis; instead, the enzyme contains sites to bind and orient catalytic cofactors. Enzyme structures may additionally contain allosteric sites wherever the binding of a tiny low molecule causes a conformational amendment that will increase or decreases activity.
A small variety of RNA-based biological catalysts referred to as ribozymes exist, which again can act alone or in complex with proteins. The most common of these is the ribosome which is a complex of protein and catalytic RNA components.
Mechanism
Substrate Binding
Enzymes should bind their substrates before they’ll catalyse any chemical change. Enzymes are usually very specific as to what substrates they bind and then the chemical reaction catalysed. Specificity is achieved by binding pockets with complementary form, charge and hydrophilic/hydrophobic characteristics to the substrates. Enzymes will so distinguish between terribly similar substrate molecules to be chemoselective, regioselective and stereospecific.
Some of the enzymes showing the best specificity and accuracy are concerned within the repetition and expression of the order. Some of these enzymes have “proof-reading” mechanisms. Here, an associate catalyst like DNA enzyme catalyses a reaction in an exceedingly beginning and so checks that the merchandise is correct in an exceedingly second step. This ballroom dance method leads to average error rates of but one error in one hundred million reactions in hi-fi class polymerases. Similar proofreading mechanisms also are found in polymer enzyme, aminoacyl tRNA synthetases, and ribosomes.
Conversely, some catalysts show enzyme sexual activity, having broad specificity and acting on a range of different physiologically relevant substrates. Many enzymes possess tiny aspect activities that arose luckily (i.e. neutrally), which may be the starting point for the evolutionary selection of a new function.
“Lock And Key” Model
To explain the discovered specificity of enzymes, in 1894 Emil Fischer projected that each the protein and also the substrate possess specific complementary geometric shapes that match precisely into each other. This is typically said as “the lock and key” model. This early model explains protein specificity but fails to explain the stabilization of the transition state that enzymes achieve.
“Induced Fit” Model
In 1958, Daniel Koshland recommended a modification to the lock and key model: since enzymes square measure rather versatile structures, the active site is continuously reshaped by interactions with the substrate as the substrate interacts with the enzyme. As a result, the substrate does not simply bind to a rigid active site; the amino acid side-chains that make up the active site are moulded into the precise positions that alter the protein to perform its chemical process perform. In some cases, such as glycosidases, the substrate molecule also changes shape slightly as it enters the active site. The situation continues to vary until the substrate is totally sure, at which point the final shape and charge distribution is determined.
Catalysis
Enzymes will accelerate reactions in many ways in which, all of which lower the activation energy (ΔG‡, Gibbs free energy)
- By stabilizing the transition state:
Creating AN surroundings with a charge distribution complementary to it of the transition state to lower its energy
- By providing an alternative reaction pathway:
Temporarily reacting with the substrate, forming a valence intermediate to supply a lower energy transition state
- By destabilising the substrate ground state:
Distorting bound substrate(s) into their transition state form to reduce the energy required to reach the transition state
By oriented the substrates into a productive arrangement to cut back the reaction entropy amendment (the contribution of this mechanism to chemical action is comparatively small)
Enzymes may use several of these mechanisms simultaneously. For example, proteases such as trypsin perform covalent catalysis using a catalytic triad, stabilise charge build-up on the transition states using an oxyanion hole, complete hydrolysis using an oriented water substrate.
Dynamics
Enzymes don’t seem to be rigid, static structures; instead they have complex internal dynamic motions – that is, movements of parts of the enzyme’s structure such as individual amino acid residues, groups of residues forming a macromolecule loop or unit of secondary structure, or even an entire protein domain. These motions make to a conformational ensemble of slightly completely different structures that interconvert with each other at equilibrium. Different states inside this ensemble could also be related to completely different aspects of AN enzymes perform. For example, completely different conformations of the protein dihydrofolate enzyme square measure related to the substrate binding, catalysis, cofactor release, and product release steps of the catalytic cycle.
Allosteric Modulation
Allosteric sites square measure pockets on the protein, distinct from the active site, that bind to molecules in the cellular environment. These molecules then cause a change in the conformation or dynamics of the enzyme that is transduced to the active site and thus affects the reaction rate of the enzyme. In this way, allosteric interactions can either inhibit or activate enzymes. Allosteric interactions with metabolites upstream or downstream in AN enzyme’s metabolic pathway cause feedback regulation, fixing the activity of the protein in step with the flux through the remainder of the pathway.
0 Comments
Submit a Comment
You must be logged in to post a comment.
CATEGORIES
- Agriculture Enzymes
- Animal Feed Enzymes
- Brewery Enzymes
- Cellulase Complex Enzymes For Cellulosic Ethanol
- Covid – 19
- Detergent Enzymes
- Disinfectants
- Distillery Enzymes
- Enzymes
- Enzymes for Biodiesel
- Enzymes For Ceramic Industry
- Enzymes for Cosmetics
- Enzymes for Crude Oil Spill Remediation
- Enzymes For Hydrolysis Of Gel Used In Crude Oil Extraction
- Enzymes for Malt
- Enzymes for soil stabilization
- Food and Beverage Enzymes
- Juice Extraction Enzymes For Wine
- Pharmaceutical Enzymes
- Pulp and Paper Enzymes
- Starch Processing Enzymes
- Sugar Processing Enzymes
- Textile Enzymes
- Wastewater Treatment Enzymes
The Basics of Soil Stabilization: An Introduction
What is Soil Stabilization? Definition: Have you ever wondered how builders manage to create rock-solid foundations for structures, even on unstable grounds? That's where soil stabilization becomes critical. At its core, soil stabilization refers to the process of...
Bio Enzyme Uses and Their Applications
Benefits of Eco Enzyme for Agriculture and Well-being
In today's world, where sustainable practices and holistic well-being are gaining prominence, the eco enzyme has emerged as a remarkable solution with numerous benefits for both agriculture and overall well-being. This powerful organic concoction offers a multitude of...
0 Comments